Each a Component in Particle Physics Equation
By Chris Bryant

One of the world’s most closely monitored experiments recently yielded its initial findings. The results, made public April 11, were made possible, in part, by three University of Alabama scientists who developed one of the primary sets of code used in key portions of the internationally-known effort’s computer analysis.
The experiment’s set-up, constructed at the Fermi National Accelerator Laboratory outside Chicago, is, to the non-scientist, bizarre. It involves a 40-foot spherical tank buried underground and filled with 250,000 gallons of baby oil, 1,520 specialized light bulbs that work in reverse, and the search for subatomic particles that, although invisible, whiz through the human body, and most everything else, by the trillions every second.
These particles, known as neutrinos, have consumed the professional life of Dr. Ion Stancu, associate professor of physics and astronomy, and UA’s lead researcher in the Fermilab experiment known as MiniBooNE.
Stancu, who has been awarded approximately $1 million in Department of Energy funding for UA’s role in the project over the last 5 years, understands why the non-physicist would be puzzled by the scientific commotion created by these electrically neutral partners of electrons.
“Neutrinos go through us, through the entire Earth, and don’t really do much,” Stancu says. “So, why the hell should we care? We do care because we want to understand the global picture of all the elementary particles, but also because there are a lot of them in the universe.”
BUNCHES AND BUNCHES
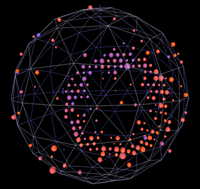
When Stancu says “a lot,” he means it. The sun, alone, produces neutrinos that bombard each square centimeter of the earth’s surface at the rate of 80 million per second. “All burning stars are putting out a tremendous flux of neutrinos,” Stancu says. “Our atmosphere is under continuous bombardment of cosmic rays. These cosmic rays also produce, among other things, neutrinos.”
A recent determination that neutrinos, or “little neutral ones,” have mass has ratcheted up their importance. “If they do have even a tiny little mass, because they are so numerous, they can have a significant contribution to the amount of mass in the universe,” the UA physicist says.
Stancu, and his UA colleagues, Dr. Yong Liu, an assistant research physicist, and Denis Perevalov, a graduate student, are among the 77 physicists from 17 institutions collaborating on the Fermilab project.
The project, some 10 years in the making, was developed to resolve questions surrounding results from a 1995 experiment at the Los Alamos Liquid Scintillator Neutrino Detector, or LSND. That experiment produced surprising evidence related to neutrino “oscillation” or how neutrinos can change from one type, which physicists refer to as a “flavor,” to another. The LSND results were inconsistent with results from other experiments, Stancu said.
“The only possible explanation for those results was the existence of an unknown neutrino, a fourth neutrino,” Stancu said. “That experiment has generated thousands of articles and many theories.”
Previously, scientists were only aware of three neutrino flavors and the existence of a fourth would have required the rewriting of the current model scientists use in their attempts to understand the universe. Its confirmation would have meant, in essence, new physics.
HOLD OFF ON THAT RE-WRITE

However, the April 11 announcement, eagerly anticipated by scientists around the world, publicly refutes the earlier experiment. “Consequently, this will also have a serious impact,” Stancu said. “We are confirming that there are only three active neutrinos, and that the presence of a sterile (the proposed fourth) neutrino has been ruled out.
“This has settled a question that has been nagging the physics community for the past 10 years. This enhances our understanding of the most fundamental properties from the most fundamental particles that make up this universe as we know it. There are still many questions to be answered in the realm of neutrino oscillation.”
The UA group was responsible for one of the key parts of the analysis, the event reconstruction and particle identification component. To understand the UA researchers’ role, additional background on the experiment’s set-up might prove helpful.
A Fermilab accelerator, several miles in diameter and buried underground some 500 meters from the detector, produces beams which, after conversion, primarily consist of one flavor of neutrinos, called muon neutrinos. Knowing the beam is primarily producing muons, scientists can then calculate, based on previous experiments, how many neutrinos would be expected to oscillate or change flavors. Finding numbers and types of neutrinos far outside the calculated range, as did the LSND experiment, would require an additional explanation.

The neutrinos travel into the detector, filled with ultra pure mineral oil that’s as clear as drinking water. There, they bump into the nuclei of atoms in the oil, creating charged particles. Devices that look like overgrown light bulbs line the detector’s walls. These bulb-like tubes, some eight inches in diameter (paper plate sized), are submerged in the oil and serve as the detector’s eyes. The charged particles generate light, which is intentionally slowed by the oil and detectable by these bulbs, known as photomultiplier tubes, or PMTs.
Stancu suggests thinking of the tubes as inverse light bulbs. “With light bulbs, you put in electricity, you get light. With these, you put in light, you get electricity.”
Different reactions generated by different types of neutrinos cause the bulbs to light up in different patterns, or what the scientists call signatures. “From the apparatus, we can record how much light got here and at what time. From that, you reconstruct where the event happened and where it was going and, moreover, what type of event it was.”
All of the related electronics used in the experiment is custom-made, Stancu says, as is all of the computer code that records and analyzes this data. Two independent analyses were conducted using two different sets of custom-made code, for cross-checking purposes. One set of code was developed by the UA researchers, and the other set was developed by Princeton researchers.
SHORTEST PATH BETWEEN TUSCALOOSA AND FERMILAB
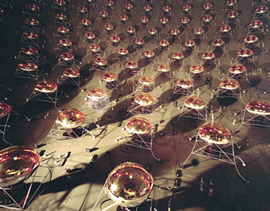
Stancu, who travels to Fermilab, on average, one weekend a month during the academic semesters, as well as spending several weeks or even months at the site during winter and summer breaks, said the experiment runs 24 hours a day, seven days per week.
“Someone has to be there constantly to watch how this is running. Everyone takes shifts, students, post-docs, professors, everyone.”
While such fundamental research might appear to be detached from every day applications, Stancu said that’s not the case.
“The methods for detecting these particles could have possible applications for non-proliferation studies,” he said. “Whenever people operate a nuclear reactor, it will always produce neutrinos. Nothing can stop those neutrinos. We can detect them and, moreover, from these neutrinos, we can learn, in principle, what kind of nuclear fuel they are burning.”
Stancu says that’s only one example, and that scientists conducting fundamental research aren’t typically tasked with developing applications. “Other people will come up with applications,” he said. “When Wilhelm Conrad Röntgen discovered x-rays, he didn’t think, ‘oh, what can I do to look through people’s bodies.’ Those applications came later. He was more interested in the phenomena of x-rays themselves.”