By Adam Jones
Acjones12@eng.ua.edu
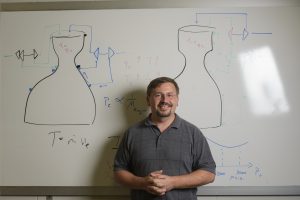
Rockets hold a unique place in our consciousness. They achieved what humans once only dreamed – escaping Earth for the heavens.
Their complexity extends beyond most people’s comprehension. We even devised ways of explaining things that did not, in fact, rely on rocket science. The opposite of rocket science is simplicity, as the saying implies.
Yet, for all the intricacy of a rocket’s design, the technology powering them has not changed much in the nearly 50 years since NASA sent men to the moon and back.
The Saturn V rocket that powered the Apollo missions in the 1960s remains the most powerful rocket ever operated. It delivered the heaviest payload humans have ever lifted off Earth, and no rocket built since has surpassed what the Saturn V could carry.
With our sights set firmly on visiting Mars, rockets need to change in a way that allows a heavier payload to be transported with a single rocket launch.
Dr. Richard Branam, a University of Alabama engineering faculty member, is working with NASA’s Marshall Space Flight Center on designing a new rocket engine that will allow rockets to take on more weight. The LOX Expansion Cycle, or LEC, rocket engine’s objective is to double the current capability for orbit transfer by increasing thrust-to-weight while maximizing performance.
“This is about increasing mass of the payload,” he says. “There’s what we can do now, and then there is the LEC technology – this engine enables new capabilities. For the same tank and structure, if we increase the performance of the engine by just 1 percent, then we increase the payload of current U.S. launch vehicles by as much as 5 percent, enough to add another transponder to a communication satellite or about 10 university satellites.”
He’s aiming for more – much more – than a 1 percent jump in performance.

Branam came to UA in 2014 after 25 years in the Air Force where he served as a researcher and engineer, retiring as a lieutenant colonel. In the Air Force, he was the principle engineer and program manager for the Upper Stage Demo Rocket Engine, a part of an initiative with the Department of Defense to double U.S. rocket propulsion capability.
That project never exceeded the demonstration phase, although Branam never gave up on the ideas hatched from that work. After arriving at UA, he was selected by NASA to work on improving portions of that concept and proving, through simulation, it could work.
Much of what is called a single “rocket” is actually several rockets stacked side-by-side or atop each other. The idea is the spacecraft can get lighter as certain tasks in the launch are finished, making it easier to continue the flight. The first stage in multi-stage rockets provides the initial thrust for liftoff before it is jettisoned from the vehicle back to Earth when its fuel tank empties, as seen in television coverage of launches.
The second stage, or upper stage, is the rocket engine that gets the vehicle into a low Earth orbit or provides the necessary escape velocity if there is a destination beyond Earth’s pull, such as the moon or Mars.
During liftoff, considerations such as the engine’s fuel economy and efficiency take a back seat to raw power. The upper stage, however, operates in a thinner atmosphere with less gravity, so it needs to work efficiently so nothing is wasted.
Branam is focused on the upper stage. While there have been upgrades to the initial technology of the Saturn V rocket, developing entirely different rockets is expensive and unfamiliar to the space community, he says. There have been new rockets, but nothing as powerful as the Saturn V.
“Although the 1960’s technology has been adapted and upgraded over the years, the upper stage propulsion system is limited by the design choices made,” Branam says.
The design choices include the use of what’s referred to as an expander cycle. It’s a closed system that uses the fuel – separated liquid oxygen and liquid hydrogen – for seemingly competing purposes. The fuel, or the propellant, both cools the rocket and is burned in combustion.
The combustion chamber inside the rocket, along with the bell-shaped nozzle seen at the bottom, has to be cooled; otherwise, it would disintegrate amidst the intense heat put out by the burning fuel fuming out.
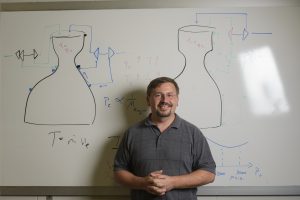
To do this, the super-cooled liquid hydrogen fuel is circulated by pumps from the fuel tanks into channels inside the walls of both the chamber and nozzle, which forms a heat exchanger. The heated fuel turns the turbines for both the fuel and oxygen pumps before being injected into the combustion chamber to burn, which ultimately provides the thrust that lifts the rocket.
“I need energy from the wall,” Branam said of the expander cycle. “If I don’t get enough energy from this heat exchange, I don’t get enough pressure. If I don’t get enough pressure, I can’t get my propellant through the cooling channels. It’s all inter-related and closed.”
This whole process has limits, theoretical and operational, that, in turn, cap what a rocket can lift outside Earth’s atmosphere. Branam’s idea is to increase the rocket’s limits through dual expander engine architecture. This LEC rocket engine concept employs two expander cycles. One pumps the fuel and the other the oxidizer.
This further separates the hydrogen and oxygen, eliminating the need for a seal between the propellants. That’s a cost savings and a decrease in manufacturing complexity. It also reduces the chance for the two elements to meet too early in the cycle, a catastrophic failure.
The LEC rocket engine pumps the hydrogen through the combustion chamber walls and then is used to turn the turbine for the fuel pump. The second expansion cycle would use the cooled liquid oxygen in the nozzle cooling channels, the bell shaped exit of the rocket engine.
The warm oxidizer will then have enough energy to turn the turbine for the oxidizer cycle. While adding complexity, a second cycle reduces the maximum power requirements for the fuel turbine. The benefit comes from not pumping fuel to the higher pressures to achieve the same performance, increasing the reliability of the pumps. The lower maximum pressure provides more operational margin and the potential to achieve higher chamber pressures.
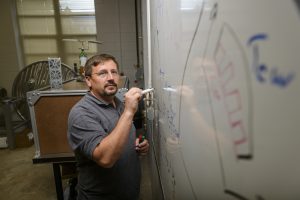
“This concept is trying to find a way to get the cycles to work together and not melt the parts. You’re cooling with the fuel and oxidizer and then you’re burning them,” he says. “You may want to burn at a slightly lower temperature, but it will obtain higher fuel economy and not melt the engine.”
NASA is well underway in developing the Space Launch System, the vehicle being designed to once again give the space agency the ability to get astronauts and other payloads to space. The LEC engine could be used in the second phase of the SLS program, taking the vehicle beyond low Earth orbit and delivering large payloads, such as a greenhouse, to Mars.
“The current technology will get about a ton of mass onto the surface of Mars,” Branam says. “The LEC engine would let them do much, much more than that – two or three times more. It’s an enabler to meet the needs of the future of spaceflight and exploration.”
Dr. Branam is a UA assistant professor of aerospace engineering and mechanics. Research reported in this article was supported by NASA/Marshall Space Flight Center under award number NNM15AA04A.