By Chris Bryant and Adam Jones

Using sunlight to create energy may seem a simple concept, but simple ideas often take creative solutions to become reality.
“It is inspired by nature, how the leaves and plants work. It is nothing but photosynthesis,” says Dr. Nitin Chopra, a nanomaterials expert at The University of Alabama.
The grade-school vocabulary word to describe the process of a plant converting the sun’s rays into fuel for itself, photosynthesis is found in even the most common leaf. Yet for its ubiquity in the natural world, humans have been unable to match the technology on a wide scale. Instead, we continue burning through fossil fuels to supply the vast majority of the energy we need, emitting environmentally harmful gasses along the way.
After decades of work that has led to success and failures, wide-spread solar energy use remains elusive. In 1979, President Jimmy Carter installed solar panels atop the White House roof. Seven years later, President Ronald Reagan ordered them removed. In August 2013, installation of solar panels began anew on the White House. Removal and subsequent return of solar energy technology atop one of the nation’s most visible landmarks mirrors the on-again, off-again momentum behind solar energy research.
INNOVATIVE SOLUTIONS

Researchers from a broad spectrum at The University of Alabama continue improving and refining solar energy, a diverse field that ranges from personal electronics to industrial-level power generation. Their work will help lead to fundamental understandings of newer solutions to a problem more than a century in the making.
Capturing and converting solar energy more efficiently are likely the world’s only long-term solution to its energy predicament of relying on a diminishing supply of fossil fuels, says Dr. David Dixon, a University of Alabama computational chemist.
When Dixon thinks about the history of solar energy, he looks back well past the 1970s “Energy Crisis” that prompted Carter’s action. And he goes right past Alexandre-Edmond Becquerel, the 19th century French physicist credited for discovering the effect by which a solar cell converts sunlight to electricity. He goes back … to the leaf.
“Plants capture solar energy that eventually gets converted in the interior of the Earth to petroleum or natural gas, and we burn those materials to release the energy stored in them,” Dixon reminds. “That’s solar energy capture that nature has done for us over a very long time. We just have to make that solar conversion a lot more efficient than it is today so we can exploit it.”

Talk with a few of the many UA researchers invested in various interdisciplinary solar energy research efforts, and you’ll hear that word, “efficiency,” time and again.
Silicon, which is processed sand, is the traditional substance used as the base material, or semiconductor, for manufacturing solar cells. Semiconductors are the materials used to turn light into an electrical current.
Silicon is abundant, but the production processes involved in producing solar panels from silicon can be expensive and energy intensive.
Solar cells made from second-generation semiconductors are cheaper to produce than those from silicon, but they are not as efficient, and their production requires the use of rare, and potentially toxic, materials, a seemingly non-sustainable option for wide-spread use.
NEW MATERIALS
Dr. Thomas Vaid, a chemistry professor and one of several University of Alabama researchers involved in solar energy-related research, is primarily interested in developing new materials that combine earth-abundant substances.
“The hope is we can tune the chemistry – try out different organic pieces with different inorganic pieces, different metals, and get things, for example, with the right electronic band gap, which is one of the fundamental parameters that determines solar cell efficiency.”
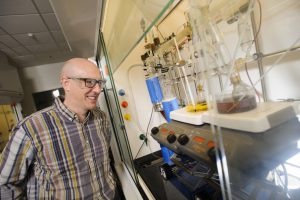
One unique material he’s developed in the lab contains carbon, sulfur and lead, and it is part of a new class of materials known as hybrid organic-inorganic semiconductors.
At the molecular level, this material Vaid synthesized contains six carbon atoms in a ring with six sulfur atoms extending from those.
Successfully substituting the sulfur atoms where, traditionally, oxygen or nitrogen atoms are found is a novel approach, and this successful atomic substitution is potentially significant for solar energy.
“If we go to sulfur instead of oxygen, it decreases the band gap, and that can make something a semiconductor. We hope to make working prototype solar cells using this material.”
Band gaps are essential in producing an electrical current. A current develops when electrons move together in the same direction. Materials with too small of a band gap produce an electric current with too little strength, or voltage.
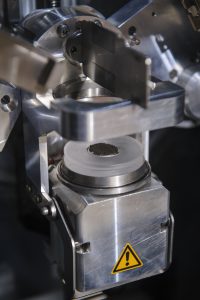
When a light particle, or photon, strikes a solar cell, electrons can become “excited” and jump from one band, or region, to another. This crossing of an adequate sized band gap produces a continual flow of electrons – an electrical current.
By substituting within the newly made material occasional atoms of different materials, in a process scientists call ‘doping,’ Vaid hopes to introduce the desired electronic properties necessary for a working solar cell.
“It will still be this material with the lead, and the sulfur and the carbon, but every one-in-a-hundred or one-in-a-thousand of these lead atoms, there will be, for example, a thallium atom there, instead.
“This actually has a pretty big effect on the electronic properties.”
Placing together two differing types of doped materials – one with a negative charge and one with a positive charge – is what makes the solar cells work.
Alternatively, Chopra is studying an application of his research into nanomaterials in seeking a new material for solar cells. One nanomaterial he works with is graphene, an atomically-thin layer of the mineral graphite useful at conducting electricity. Chopra uses semiconducting quantum dots less than 5 nanometers in size and tagged onto graphene shells encapsulating metal nanoparticles for solar light harvesting. The configuration of a nanoscale structure of two different materials has a strong potential to enhance photovoltaic properties, or the ability to get energy from the sun, using graphene-quantum dot architectures.
With “thin-film” solar cells, such as those Vaid and others are interested in producing, the semiconducting materials are deposited on a sliver of the cell’s base, which is usually glass or metal. Research in the lab of UA’s Dr. Arunava Gupta has shown that nanocrystal suspensions of copper, zinc, tin and sulfur can replace current semi-conducting materials in thin-film cells, reducing cost.
NEW METHODS
But more than switching materials, Gupta is working on a method of using cheaper, more common materials and spraying them on glass like spray paint on a wall to create a cell. This fabrication method is easier and considerably cheaper than the current method of making thin-film cells.
Another type of thin-film cell is a dye sensitized solar cell that is easier to fabricate, non-toxic and works at lower light levels. They are presently the least efficient of all cells, but the cheapest to make. They could provide electricity in applications where more expensive cells would negate the benefits of solar energy.

Dye sensitized solar cells rely on the noble metal platinum that could be swapped to lower costs. In this case, platinum is not a semiconductor. It is a catalytic counter electrode, meaning it uses some of the electricity in the cell to regenerate the dye, or electrolyte solution, on the cell that the sun’s rays excite to create electricity. Swapping platinum for a low-cost, earth-abundant nanocrystal film coating of copper, zinc, tin and sulfur would also improve the cell’s efficiency, according to research results in Gupta’s lab.
A CELL WITH FLEXIBILITY
Though limited, thin-film cells available commercially are still inorganic like their big brother, the traditional silicon cell. However, emerging organic polymer solar cells are made of a thin film, making them more flexible, lighter and cheaper to produce. Able to be printed roll-to-roll like a newspaper, they have great potential for large-scale production.
This emerging type of solar cell could initially replace traditional solar energy technology in niche applications such as powering a house by taping flexible organic solar cells onto windows or the roof or to recharge small consumer electronics in a car through a flexible sun-blocking shield of solar cells. Another interesting application could be for flexible displays. Travelers could carry a rolled/folded electronic map, with polymer solar cells integrated on their clothes to power the map.
Although there has been dramatic progress with energy conversion in organic polymer solar cells, they are about half as efficient at capturing the sun’s energy as silicon solar cells. They also don’t last as long. To address the efficiency and the reliability issues from polymer solar cells, Dr. Dawen Li, an expert in organic electronic devices, proposes making high-performance solar cells with a novel bilayer structure. The polymer brushes in the bottom layer provide direct pathways for electric current, while the top-blend layer with a copolymer would allow for better harvesting of the sun’s energy.
“The research outcome will enrich current understanding of the fundamental structure-property relationships involved in polymer photovoltaics and drive polymer solar cells to become economically competitive in the near future,” Li said.
OPTICAL SIGNALS
Seeking a better understanding, at the molecular level, of how organic solar cells work is Dr. Shanlin Pan, a professor in UA’s department of chemistry.

For the organic solar cells to generate electricity, the charges must be persuaded to move quickly enough across the organic thin film. Researchers refer to this issue as charge transport. Another challenge is the material’s lack of stability.
Taking tiny cross sections from organic solar cells and placing them under powerful, specialized microscopes, Pan and his collaborators send and withdraw voltage into the samples while observing and measuring, at the molecular level, how the charge transport fails and where the material’s molecules lose stability.
“If we can use our electrochemistry spectroscopy tools to study that molecule and understand its behavior in the solar cell at the nanometer scale, we can back calculate what could be done to improve that device’s efficiency,” Pan said.
As electrons move across the band gap, the organic materials either absorb or emit light, and these UA researchers watch this unfold. By measuring changes in the molecules’ optical signals – including their intensity and wavelengths – the researchers gain a better understanding of some of the “whys” behind the solar cells’ shortcomings.
In Pan’s lab, this involves the use of a special microscopic set-up, one designed by the UA researcher. This spectroelectrochemistry microscope has resolution capabilities at the nanometer scale. (A nanometer, to better envision the capabilities of this instrument, is about 7,000 times smaller than a red blood cell.)
The instrument can also reveal how the particles within the material are distributed and how that distribution changes in difference circumstances.
In collaboration with researchers at Emory, Notre Dame and Michigan State, the group also uses ultra fast laser spectroscopy to measure the speed at which the charge transport occurs. In addition to UA doctoral students and a post-doctoral researcher, the UA chemist also collaborates with UA’s Dixon and two other campus colleagues, Gupta and Dr. Shane Street.
ONLY AS GOOD AS WEAKEST CELL
Solar technology can also be improved through the electronic systems that convert the energy in the cell into useful electricity.
“In a lab, you can test a cell’s potential, but, in real life, you have to control it in such a way that it gets that efficiency under varying conditions and when powering a real load,” says Dr. Jaber Abu-Qahouq, an electrical engineering professor.
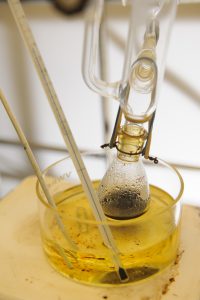
Abu-Qahouq works to improve the system architecture and controls of photovoltaic solar systems. Solar cells are connected with electronics that process electric power and controls that ensure maximum power is extracted and delivered in an appropriate form to loads. Research in Abu-Qahouq’s lab has led to developments that could reduce the amount of electronics and controls needed to operate closer to maximum power point, reducing cost and size while improving efficiency, in solar systems with advanced architectures.
A challenge for solar arrays is mismatching conditions. Like a car with one bad tire reducing fuel efficiency and safety, a solar array is only as good as the weakest cell.
A way around this problem is a distributed system where each panel, or even each cell, has its own electronics to process its energy, but that means adding significant size and cost. Using intelligent algorithms with associated power electronics, Abu-Qahouq’s system reduces a distributed system control hardware to one controller and one sensor, getting it closer to a non-distributed system’s size and cost of control and sensing, but with higher efficiency. A patent is already pending on the technology.
STORE NOW, USE LATER
Another type energy, solar thermal energy, could be a replacement for many industrial-scale power production technologies.
It uses mirrors to focus the heat of the sun on a liquid that, in turn, heats up water that vaporizes into steam to turn power generators.
One powerful advantage of solar thermal energy is the ability to store the heating liquid for use at night or on cloudy days. In essence, it is storing the energy needed to make electricity.
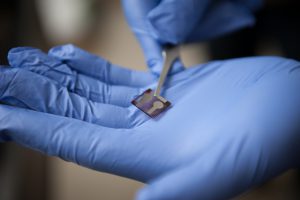
“The future is going to be energy storage and using it later,” says Dr. Ramana Reddy, a UA metallurgy expert.
By tinkering with the chemical composition of molten salts, Reddy can hold off freezing – a common problem with the salts – and increase how many hours the molten salt can be stored as a heating liquid for use in power generation.
Reddy has a goal of increasing storage 12 to 18 hours, two to three times longer than current, commercially-available molten salts. This could lower costs.
A CRITICAL JUNCTURE
By using computers to predict the properties of molecules, Dixon, the computational chemist, supports multiple UA solar-powered research efforts.
He says the nation is approaching a critical juncture in its solar research.
“I think there is a huge amount of momentum behind it right now,” Dixon says. “The U.S. has to make sure it doesn’t let the whole solar power business end up elsewhere. We have to have a major role in it. We don’t want to have to rely on other countries to provide us with that kind of technology or we will end up in the same place we are with oil. If somebody else is providing that technology to you, then they control you and your economy.”
Dr. Dixon is a professor of chemistry and the Robert Ramsay Chairholder, while Drs. Pan and Vaid are assistant professors of chemistry and Dr. Street is an associate professor of chemistry, all in the College of Arts and Sciences. Dr. Gupta is a Distinguished University Research Professor with appointments in chemistry and chemical and biological engineering. Dr. Abu-Qahouq is an associate professor while Dr. Li is an assistant professor, both in electrical and computer engineering, Dr. Chopra is an associate professor of metallurgical and materials engineering, and Dr. Reddy is the ACIPCO Professor of Metallurgical and Materials Engineering. Supporters of aspects of this research include the U.S. Department of Energy, the National Science Foundation, the U.S. Department of Agriculture and Alabama NASA EPSCoR.